Background: Epidemiological studies have shown that sex hormone-binding globulin (SHBG) has a role in glucose homeostasis in both men and women. However, a prospective study on Japanese-American subjects concluded that SHBG was not a significant risk factor in either men or women, suggesting ethnic differences. We were not aware of any evaluation of SHBG in subjects of African ancestry.Objectives: We investigated the association between SHBG and insulin resistance in type 2 diabetic diabetic men in a hospital in Nigeria. Method: Forty-eight male subjects with type 2 diabetes and 20 non-diabetic male subjects were recruited in this cross-sectional hospital-based study by the convenient sampling method. Height and circumferences around the waist and hip were measured to the nearest 0.5 cm and the waist–hip ratio was calculated from this measurement. Weight was measured and body mass index was calculated. Fasting plasma glucose concentration was measured by the glucose oxidase method with a between-run coefficient of variation of 3%. Insulin and SHBG were measured by means of enzyme-linked immunosorbent assay (ELISA). Results: There was a statistically-significant difference between test results for the diabetic and non-diabetic patients. The mean SHBG concentration was higher in the non-diabetic group (42.2 nmol/L) than the diabetic group (30.5 nmol/L). A significant inverse association between insulin resistance and SHBG was observed (r = 0.353, p < 0.015). Conclusion: This study supported earlier observations that a significant inverse correlation exists between SHBG and insulin resistance and provides evidence that the relationship may extend to type 2 diabetic men of African ancestry in Nigeria.
Diabetes mellitus has been recognised as a disease for more than 3500 years, since its description in the Eber Papyrus in 1552BC.1 Surviving texts from the ancient Greeks, Chinese, Indians, and Persians also refer to the disease, which was subsequently named diabetes mellitus. It is defined as a metabolic disorder of multiple aetiologies, characterised by chronic hyperglycaemia with disturbance of carbohydrate, fat, protein, water and electrolyte metabolism which result from defects in insulin secretion, insulin action or both.2 Much of the morbidity and mortality burden of diabetes mellitus is due to the acute and chronic complications that often arise. These complications include damage to various organs, especially the eyes, kidneys, nerves, heart and blood vessels. In Nigeria, 3.9 million people have diabetes mellitus, representing an estimated prevalence
rate of 4.04%.3 Although laboratory tests to screen for and diagnose diabetes
mellitus are widely available, the disease is still underdiagnosed. Approximately 25% of people with
newly-diagnosed diabetes mellitus already have microvascular disease, suggesting that they had the disease for four to seven years by the time of diagnosis.4,5Because there are no unique biological markers and few symptoms in the early years of the disease, the diagnosis of diabetes mellitus is based exclusively on hyperglycaemia the consequence of a disrupted carbohydrate metabolism.
The tests for early diagnosis of diabetes mellitus are limited to direct and indirect tests of hyperglycaemia namely, serum
glucose and glycated protein (HbA1c), respectively. Serum glucose testing is affected by short-term lifestyle changes, lower
reproducibility and sample processing6 (e.g. during the oral glucose tolerance test, incretin effect
on the ingested glucose cannot be accounted for, neither can it be standardised, which affects reproducibility). The use of HbA1c
for screening for and diagnosing diabetes mellitus also has its limitations, as it is only able to reflect glycaemic control in retrospect and is
also affected by haemolytic anaemia.7,8,9,10,11,12,13,14 It therefore follows that a new test that can identify type 2 diabetes mellitus earlier, with greater sensitivity and specificity, will be desirable. An emerging alternative is sex hormone-binding globulin (SHBG). Sex hormone-binding
globulin, once considered to be merely a way for the body to store extra sex hormones, now appears to be a player in a
number of biological functions. There is evidence showing a more direct role for SHBG through an intracellular signaling
cascade mediated by a membrane-bound SHBG receptor.15,16 Delineation
of the biological effect of SHBG signaling has lagged behind our understanding of the biochemical analysis of its cAMP
signaling pathway; however, epidemiological studies on subjects of European ancestry have reported an inverse association
between low circulating SHBG and type 2 diabetes.17,18 This means
that those with higher serum levels of SHBG are protected from developing type 2 diabetes. Genetic studies have defined
a causal relationship between SHBG and type 2 diabetes mellitus. Genetic studies have defined a causal relationship between
SHBG and type 2 diabetes mellitus in subjects of European ancestry. In a prospective study on Japanese- in subjects of
European ancestry. In a prospective study on Japanese-American subjects who were followed for 3 years, however, SHBG did
not correlate with insulin resistance in either men or women,19 suggesting ethnic differences.
There is a paucity of data on the relationship of SHBG with type 2 diabetes mellitus in subjects of African ancestry.
This study investigated the association between circulating SHBG and type 2 diabetes in in a group of Nigerian men.
Research method and design
|
|
Participants
This cross-sectional study was conducted at University College Hospital, Ibadan, Oyo State, Nigeria. The formula Zα2ε2/d2 was used to determine the sample size, where Zα is the standard normal deviate of α at 5%, ε is the standard deviation of 9.11 as defined by a previous study17 and d is the level of precision. Substituting 1.96 for Zα and 3 for d in the formula gives a sample size of 34.5. Adding a non-response rate of 10% brings the required sample population to approximately 40. Forty-eight male participants with type 2 diabetes mellitus and 20 controls, were recruited from the General Outpatient Department, Metabolic Research Clinic and Medical Outpatient clinics in University college Hospital by the convenient sampling. All adult men diagnosed with type 2 diabetes mellitus
who attended the clinics during 2011 were invited to participate. The response rate was 62%. None were turned away, which led
to a sample size that was greater than initially called for. These were Nigerians residing in Ibadan, the western part of
Nigeria. Exclusion criteria included injured and very sick, those on medication such as insulin, steroids, anticonvulsants,
beta blockers and thiazides. Informed consent was obtained and the study lasted for the year of 2011.
Collection of blood and clinical data
Seven millilitres of fasting venous blood were drawn from the antecubital vein between 08h00–09h00 and aliquoted into fluoride oxalate tubes for glucose and plain tubes for hormonal (insulin and SHBG) assays. The plain tube bloods were allowed to clot for 30 minutes and the sera were collected after centrifugation at 4500 rpm for five minutes, then stored at 80 °C for four months before analysis. Waist (iliac crest) and hip (widest region at the tronchanter level) circumference were measured to the nearest 0.5 cm and from these measurements, the waist–hip ratio (WHR) was calculated. Weight was measured in kilograms using a weighing balance (Medical Laboratory Scientific England). A Stadiometer RGZ 160 (Medical Laboratory Scientific England) was used to measure height, from which body mass index (BMI) was calculated (kg/m2). Biodata and self-reported drug history were also documented.
Laboratory analysis
Plasma glucose concentration was measured by the glucose oxidase method, using a PUS 2018G Biochemistry Analyzer (Beijng Perlong New Technology and Co Ltd, China) with a between-run coefficient of variation of 3% (glucose concentration of 4.94 mmol/L at 10 replicates). Insulin and SHBG concentration were measured using enzyme-linked immunosorbent assay (ELISA) kits (GenWayBio, San Diego, CA), according to the kit protocol. Briefly, these are solid phase enzyme-amplified heterogeneous immunoassays performed on microtiter plates.20 ELISA technology uses monoclonal antibody (Mab) directed against distinct epitopes. Calibrators and samples react with the capture monoclonal antibody (Mab 1) coated on the microtiter well and with monoclonal antibody (Mab 2) labeled with horseradish peroxidase. After an
incubation period allowing the formation of a sandwich (Mab 1 target Mab 2 horseradish peroxidase, where target, in this case, is human insulin or SHBG), the microtiter plate is washed to
remove unbound enzyme-labeled antibody. A chromogenic solution (tetramethylbenzydine) is added and incubated. The reaction is stopped and the absorbance, which is
directly proportional to the insulin or SHBG concentration, is measured. A calibration curve is plotted using 5-parameter logistic software (www.readerfit.com)
and the insulin or SHBG concentration of the samples is interpolated from the calibration curve. The insulin ELISA kit had an interassay coefficient of variation of 13% (kit controls for insulin concentration set at 25 µU/ml [control 1] and 80 µU/ml [control 2] at 5 replicates) and 0.3% cross-reactivity with
proinsulin; and the SHBG kit had an interassay coefficient of variation of 16% (SHBG concentration = 50 nmol/L at 9
replicates). Fasting insulin and glucose values were used to calculate insulin sensitivity, B-cell function and insulin
resistance using the mathematical equation described by Jonathan Levy et al. in 1998,21 as calculated
using HOMA-2 Calculator software (www.dtu.ox.ac.ukM).
Statistical analysis
The data were log transformed to enhance compliance with normality assumptions. One observed value for SHBG was treated as an outlier using the Dixon test.22 The Dixon test uses the ratio D/R, where D is the absolute difference between an extreme observation (large or small) and the next largest and R is the range between the largest and smallest observations, including extremes. The cut-off value for the ratio (D/R) is 1/3; that is to say, if the observed value for D is equal to or greater than one third of the range R, the observed value is deleted. Mean and standard deviation (SD) were calculated for anthropometric characteristics and laboratory-measured and -derived data (age, BMI, WHR, fasting glucose, fasting insulin, SHBG, insulin resistance, insulin sensitivity and pancreatic beta-cell function). Simple linear regression and
Mann-Whitney U-tests were also performed. The Statistical Package for Social Science 16.0
software was used for the computation.
The mean age was similar for the diabetics (62, SD = 11) and the controls [non-diabetics] (60, SD = 14) (Table 1).
Twenty-five (52.5%) of the diabetic subjects had a normal BMI whilst 14 (25%) were overweight, 8 (20%) were Class I obese
and 1 (2.5%) were Class II obese. Twenty-seven subjects (56.3%) had a normal WHR of < 1 and within this group, 17 (63%)
had a normal BMI. In the control group, 10 (50%) subjects had normal BMI, 6 (30%) were overweight and 4 (20%) were class
I obese. WHR was < 1 except for 3 (15%) that were > 1. Within the abnormal WHR control group, 2 (10%) were overweight and 1 (5%) class I obese.
There was no local reference range for SHBG so the kit manufacturer’s reference interval of 15–100 nmol/L was used to interpret the results. There was a statistically-significant difference between the means of SHBG concentration in the controls and the diabetic group (Mann-Whitney U = 307, p = 0.026, 2-tailed). In the diabetic group, the SHBG level of 36 (75%) subjects was within the reference range, whilst 11 (23%) had high values (above the upper limit of the reference interval) and only one subject had an abnormally low value (below the lower limit). Five (25%) subjects in the control group had SHBG levels above the upper limit of the reference interval whilst the others were within the reference range. The mean fasting insulin concentration was 13.64 μU/ml in the diabetic group and 12.34 μU/ml in the controls, with no statistically-significant difference being observed. Forty (83%) subjects had fasting insulin concentrations within the kit manufacturer’s reference range of 5–19 µU/ml, one had a result that fell below the lower limit of the reference interval and seven (15%) had values above the upper limit. Two (10%) subjects in the control group had insulin concentration above the reference interval whilst 18 (90%) were within the interval. A weak (though statistically significant) inverse association (r = 0.353, p < 0.015) was observed between insulin
resistance and SHBG (Figure 1), whereas a positive association (r = 0.356, p < 0.014)
was observed between age and circulating SHBG (Figure 2). There was no significant correlation between SHBG and
fasting insulin (r = 0.264, p < 0.073) or between SHBG and fasting
glucose (r = 0.236, p < 0.110). There was no significant correlation
between SHBG and BMI (r = 0.189, p < 0.203) or between SHBG and
WHR (r = 0.126, p < 0.397). Similarly, IR did not correlate
significantly with insulin resistance (r = 0.043, p < 0.772)
or with WHR (r = 0.068, p < 0.646).
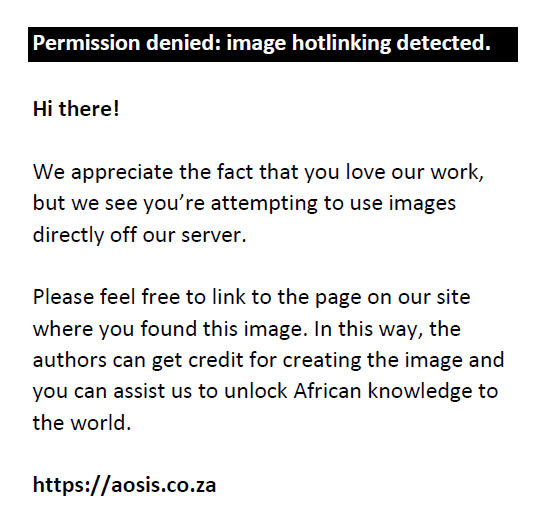 |
FIGURE 1: Scatter plot showing the relationship between insulin resistance and
levels of sex hormone-binding globulin.
|
|
 |
FIGURE 2: Scatter plot showing the relationship between age and levels of sex
hormone-bindin.
|
|
Ethical Approval was given by the Institute for Advanced Medical Research and Training, University of Ibadan, Nigeria, with UI/UCH EC Registration Number: NHREC/05/01/2008a.
Potential benefits and haz
There was no potential physical, psychological or disclosure danger to the subjects except the pain of the needle-prick when the blood samples were taken.
Recruitment procedures
Participation was voluntary and subjects were free to withdraw at any time. This entitlement lapsed on 15 December 2011, at the conclusion of the study.
Informed consent
Informed written consent was signed by each participant.
Data protection
Each participant was assigned a number which was known only to him and the investigator. These numbers were used for blood sample collection so the technologist did not know whose sample was being analysed.
Reliability
All assays were performed in duplicate. Glucose estimation by the glucose oxidase method had a between-run coefficient of variation of 3% (glucose concentration of 4.94 mmol/L at 10 replicates). The insulin estimation had an interassay coefficient of variation of 13% (kit controls for insulin concentration set at 25 µU/ml [control 1] and 80 µU/ml [control 2] at 5 replicates) and 0.3% cross-reactivity with proinsulin; and the SHBG kit had an interassay coefficient of variation of 16% (SHBG concentration = 50 nmol/L at 9 replicates).
There are four important findings that can be drawn from this study:1. We found a weak inverse correlation between the serum concentration of SHBG and insulin resistance amongst our group of Nigerian men with type 2 diabetes. 2. We identified a statistically-significant difference between the concentration of SHBG in diabetic and non-diabetic men. 3. There was no significant correlation between insulin resistance and BMI/WHR amongst type 2 diabetic men in this study. 4. SHBG did not correlate meaningfully with fasting insulin and fasting glucose amongst these subjects. There is a paucity of studies on the relationship between SHBG and type 2 diabetes in men of African descent. In the 1990s Birkeland et al.17 observed a direct correlation between insulin sensitivity and SHBG levels in men of European ancestry which was independent of obesity and abdominal fat accumulation. They estimated insulin sensitivity using the hyperinsulinaemic euglycaemic glucose clamp (the gold standard test) and fat distribution with BMI and WHR. Haffner et al.,18 in their nested case control study, observed a consistent inverse correlation between SHBG concentration at baseline and the subsequent development of diabetes in men. The findings in this study are in agreement with their findings, in that we also found an inverse correlation between SHBG and insulin resistance. Association does not mean causality, but two recently-published studies using a Mendelian randomisation approach provided strong evidence that
circulating SHBG is involved directly in the pathogenesis of type 2 diabetes in both men and women.24,25 These studies described the effect of genotypes of gene-encoding SHBG on plasma levels of SBHG and the risk of development of type 2 diabetes. In these nested case control studies, gene analysis of the single nucleotide polymorphisms (SNPs), rs1799941, rs6257 and rs6259, coding for circulating SHBG, was performed in men and women with type 2 diabetes and matched with controls, indicating that low levels of SHBG are a strong predictor for the development of type 2 diabetes mellitus. Since genetic studies are a powerful epidemiological tool, their findings may now help to more deeply address the complex and indubitably important role of circulating SHBG in the natural history of type 2 diabetes mellitus. The current study was able to replicate some of the previously-mentioned findings in subjects. Circulating SHBG had an inverse correlation with insulin resistance. Surprisingly, no significant association between insulin resistance and BMI/WHR was observed, even though they are strong predictors for the risk of developing type 2 diabetes,26,27,28 probably because of the complex interaction of genetics, environment and lifestyle choices in the aetiology of the disease. It was also observed that there was no significant association between body fat distribution (BMI and WHR) and circulating SHBG, which is in agreement with earlier observations that circulating levels of SHBG are determined by liver fat and not total body fat.29 Insulin decreases the hepatic production of sex hormone-binding globulin in vitro,30 meaning
that inhibition of insulin release increases plasma levels of sex hormone-binding globulin in humans.31 Hyperinsulinaemia induced by insulin resistance probably causes low levels of SHBG in insulin-resistant conditions such as obesity, type 2 diabetes and polycystic ovary syndrome. Interestingly, no correlation between circulating SHBG and fasting insulinaemia did not reach statistical significance at the 0.05 level. The findings in this study argue against the widely-held explanation that hyperinsulinaemia is responsible for a decline in circulating SHBG in humans.31 The positive correlation between age and SHBG levels supports earlier finding that SHBG levels increase once people are in their sixties.32,33 This implies that the elderly should be protected from the development of type 2 diabetes mellitus but,
paradoxically, age is a risk factor for development of the disease and screening is recommended from the age
of 45 years.34 This would seem to argue against this observation, although the explanation could be that those that eventually develop type 2 diabetes mellitus are genetically disposed toward having low circulating SHBG levels, as was suggested by Ding et al.25 The statistical difference between the SHBG concentration in the two groups suggests that SHBG estimation could be useful in the management of type 2 diabetes. Taken together, these findings indicate that a significant inverse association exists between circulating SHBG and insulin resistance.
Measurement of insulin resistance was one of the recognised difficulties in this study. Insulin resistance was calculated using an indirect measurement (a surrogate index using the HOMA 2 calculator) which has a significant but imperfect correlation (r = 0.88, p < 0.0001)35 with the
direct gold-standard measurement (the hyperinsulinaemic euglycaemic clamp method) of insulin resistance.
BMI and WHR were used as proxies for a more direct assessment of overall adiposity.
Recommendations
Evidence for the underlying mechanism behind the association between SHBG levels and insulin resistance is yet to be elucidated fully. Despite the association between SHBG and type 2 diabetes, further studies to evaluate changes in sex hormone-binding globulin concentration as glycaemic control improves, as well as delineation of the underlying mechanism regarding how SHBG affects glucose homeostasis, are necessary as they may provide novel targets for diabetes prevention and management.
This study supports earlier observations that a significant, though weak, inverse correlation exists between SHBG and insulin resistance and extends to type 2 diabetic Nigerian men. Further studies to delineate the underlying mechanism behind the association between SHBG and type 2 diabetes mellitus are advocated as they may provide a novel target for prevention and management of type 2 diabetes mellitus.
Special thanks to Dr Elizabeth Luman and her team for their editing assistance.
Competing interests
The authors declare that they have no financial or personal relationship(s) that may have inappropriately influenced them in writing this article.
Authors’ contributions
F.M.A. ( University College Hospital), A.N.A. (University College Hospital), O.O.O. (University College Hospital), confirmed they have contributed equally to the intellectual content of this paper and have met the following three requirements: (a) significant contributions to the conception and design, acquisition of data, or analysis and interpretation of data; (b) drafting or revising the article for intellectual content; and (c) final approval of the article.
1. Carpenter S, Rigard M, Barile M, et al. An interlinear transliteration and English translation of portions of The Ebers Papyrus. New York: Annadale-on-Hudson; 1998.2. World Health Organization. Definition, Diagnosis and Classification of Diabetes Mellitus and its Complications. [page on Internet]. [c2006] [cited 2011 Jan 30].
Available from http://apps.who.int/iris/bitsteam/10665/66040/1/WHO_NCD_NCS_99.2.pdf 3. International Diabetes Federation. IDF diabetes atlas. [homepage on Internet] [n.d.] [cited 2011 Jan 30]. Available from http://www.idf.org 4. Harris MI. Undiagnosed NIDDM: clinical and public health issues. Diabetes Care. 1993;16(4):642–652. PMid:8462395
5. Harris MI, Klein R, Welborn TA, et al. Onset of NIDDM occurs at least 4–7 yr before clinical diagnosis. Diabetes Care. 1992;15(7):815–819.
http://dx.doi.org/10.2337/diacare.15.7.815, PMid:1516497
6. Cox ME, Edelman D. Test for screening and diagnosis of Type 2 Diabetes. Clin Diabetes. 2009;27(4):132–138.
http://dx.doi.org/10.2337/diaclin.27.4.132 7. Selvin P, Crainiceanu CM, Brancati FL, et al. Short-term variability in measures of glycemia and implications for the classification of diabetes. Arch Intern Med. 2007;167(14):1545–1551.
http://dx.doi.org/10.1001/archinte.167.14.1545, PMid:17646610
8. Pani LKL, Meigs J, Driver C, et al. Effect of aging on A1C levels in individuals without diabetes: Evidence from the Framingham Offspring Study and the National Health and Nutrition Examination Survey 2001–2004. Diabetes Care. 2008;31(10):1991–1996.
http://dx.doi.org/10.2337/dc08-0577, PMid:18628569, PMCid:2551641 9. Herranz LS, Grande C, Pallardo LF. Non-glycemic-dependent reduction of late pregnancy A1C levels in women with type 1 diabetes. Diabetes Care. 2007;30(6):1579–1580.
http://dx.doi.org/10.2337/dc06-2568, PMid:17363748
10. Gama R, Likhari T. Diagnosis of diabetes. Haemoglobin A1c: ethnic differences apply to the UK. BMJ. 2009;339:b5648.
http://dx.doi.org/10.1136/bmj.b5648, PMid:20044400
11. El-Agouza I, Abu SA, Sirdah M. The effect of iron deficiency anaemia on the levels of haemoglobin subtypes: possible consequences for clinical diagnosis. Clin Lab Haematol. 2002;24(5):285–289.
http://dx.doi.org/10.1046/j.1365-2257.2002.00464.x, PMid:12358889
12. Szymezak JLE, Martin M, Leroy N, et al. Carbamylated hemoglobin remains a critical issue in HbA1c measurements. Clin Chem Lab Med. 2009;47(5):612–613.
http://dx.doi.org/10.1515/CCLM.2009.136, PMid:19397488
13. McCance DR, Hanson RL, Charles MA, et al. Comparison of tests for glycated Hemoglobin and fasting and 2 hours plasma glucose concentration as diagnostic methods for diabetes. BMJ. 1994;308(6940):1323–1328.
http://dx.doi.org/10.1136/bmj.308.6940.1323, PMid:8019217, PMCid:2540244 14. Coban E, Ozdogan M, Timuragaoglu A. Effect of iron deficiency anemia on the levels of hemoglobin A1c in nondiabetic patients. Acta Haematol. 2004;112(3):126–128.
http://dx.doi.org/10.1159/000079722, PMid:15345893
15. Kahn SM, Hryb DJ, Nakhla AM, et al. Sex hormone binding globulin is synthesised in target cells. J Endocrinol. 2002;175(1):113–120.
http://dx.doi.org/10.1677/joe.0.1750113, PMid:12379495
16. Hammes A, Andreassen TK, Spoelgen R, et al. Role of endocytosis in cellular uptake of sex steroids. Cell. 2005;122(5):751–762.
http://dx.doi.org/10.1016/j.cell.2005.06.032, PMid:16143106
17. Birkeland K, Hanssen K, Torjesen P, et al. Level of sex hormone-binding globulin is positively correlated with insulin sensitivity in men with type 2 diabetes. J Clin Endocrinol Metab. 1993;76(2):275–278.
http://dx.doi.org/10.1210/jc.76.2.275, PMid:8432768
18. Haffner SM, Shaten J, Stern MP, et al. Low levels of sex hormone-binding globulin and testosterone predict the development of non-insulin-dependent diabetes mellitus in men. Am. J. Epidemiol. 1996;143(9):889–897.
http://dx.doi.org/10.1093/oxfordjournals.aje.a008832, PMid:8610702
19. Okubo M, Tokui M, Egusa G, et al. Association of sex hormone binding globulin and insulin resistance among Japanese-American subjects. Diabetes Res Clin Pract. 2000;47(1):71–75.
http://dx.doi.org/10.1016/S0168-8227(99)00106-0 20. Kaplan LA, Pesce AJ, Kazmierczak SC. Clinical chemistry: theory, analysis, correlation. 3rd ed. Maryland Heights: Mosby. 21. Levy JC, Matthews DR, Hermans M. Correct homeostasis model assessment (HOMA) evaluation uses the computer program. Diabetes Care. 1998;21(12):2191–2192.
http://dx.doi.org/10.2337/diacare.21.12.2191, PMid:9839117
22. Dixon W. Processing data for outliers. Biometrics. 1953;9(1):74. http://dx.doi.org/10.2307/3001634 23. World Health Organization. BMI classification. Global database on Body Mass Index. [page on Internet] [c2006] [cited 2011 Aug 30]. Available from http://www.who.int/bmi/index.jsp?introPage=intro13.html 24. Perry JR, Weedon MN, Langenberg C, et al. Genetic evidence that raised sex hormone binding globulin (SHBG) levels reduce the risk of type 2 diabetes. Hum Mol Genet. 2010;19(3):535–544.
http://dx.doi.org/10.1093/hmg/ddp522, PMid:19933169, PMCid:2798726 25. Ding EL, Song Y, Manson JE, et al. Sex hormone-binding globulin and risk of type 2 diabetes in women and men. N Engl J Med. 2009;361(12):1152–1163.
http://dx.doi.org/10.1056/NEJMoa0804381, PMid:19657112, PMCid:2774225 26. American Diabetes Association. Standard of medical care in diabetes–2010. Diabetes Care. 2010;33(Suppl 1):S11–S61.
http://dx.doi.org/10.2337/dc10-S011, PMid:20042772, PMCid:2797382 27. Haffner SM, Stern MP, Mitchell BD. Incidence of type II diabetes in Mexican Americans predicted by fasting insulin and glucose levels, obesity, and body fat distribution. Diabetes. 1990;39(3):283–288.
http://dx.doi.org/10.2337/diabetes.39.3.283, PMid:2407581
28. Lundgren H, Bengtsson C, Blohme G, et al. Adiposity and adipose tissue distribution in relation to incidence of diabetes in women: results from a
prospective population study in Gothenburg, Sweden. Int J Obes. 1989;13(4):413–423. PMid:2793297
29. Selva DM, Hogeveen KN, Innis SM, et al. Monosaccharide-induced lipogenesis regulates the human hepatic sex hormone-binding globulin gene. J Clin
Invest. 2007;117(12):3979–3987. PMid:17992261, PMCid:2066187 30. Plymate SR, Matej LA, Jones RE, et al. Inhibition of sex hormone binding globulin production in the human hepatoma (Hep G2) cell line by insulin and prolactin. J Clin Endocrinol Metab. 1988;67(3):460–464.
http://dx.doi.org/10.1210/jcem-67-3-460, PMid:2842359
31. Nestler JE, Powers LP, Matt DW, et al. A direct effect of hyperinsulinemia on serum sex hormone-binding globulin levels in obese women with the polycystic ovary syndrome. J Clin Endocrinol Metab. 1991;72(1):83–89.
http://dx.doi.org/10.1210/jcem-72-1-83, PMid:1898744
32. Anderson DC. Sex-hormone-binding globulin. Clin Endocrinol (Oxf.). 1974;3(1):69–96.
http://dx.doi.org/10.1111/j.1365-2265.1974.tb03298.x 33. Vermeulen A. Physiology of the testosterone-binding globulin in man. Ann N Y Acad Sci. 1988;538:103–111.
http://dx.doi.org/10.1111/j.1749-6632.1988.tb48855.x, PMid:3056178
34. Sacks DB, Arnold M, Bakris GL, et al. Guidelines and recommendations for laboratory analysis in the diagnosis and management of diabetes mellitus. Diabetes Care. 2011; 34(6):e61–99.
http://dx.doi.org/10.2337/dc11-9998, PMid:21617108, PMCid:3114322 35. Wallace TM, Levy CJ, Matthew RD. Use and abuse of HOMA modeling. Diabetes Care. 2004;27(6):1487–1495.
http://dx.doi.org/10.2337/diacare.27.6.1487, PMid:15161807
|